In the first half of the 20th century, developmental biology and genetics were separate disciplines. The term epigenetics was coined in 1942 by developmental biologist Conrad H. Waddington to link the two fields. "Epi-" (a Greek prefix meaning “upon, over, on top of”) + “genetics” = “on top of genetics”.
Epigenetics, essentially, affects how genes are read by cells, and subsequently, how they produce proteins. Technically, it is the study of mitotically heritable yet potentially reversible, molecular modifications to DNA and chromatin without alteration to the underlying DNA sequence.
Certain circumstances in life can cause genes to be silenced or expressed over time. In other words, they can be turned off (dormant) or turned on (active). Basically, epigenetics is the study of biological mechanisms that will switch genes on and off or “modify” genes. Hence, modifications in gene expression that are independent of the DNA sequence of a gene are called epigenetic alterations.
This modification of gene expression may affect numerous biochemical dysregulations and dysfunctions. These alterations may contribute to epigenetic inheritance and epigenetic carcinogenesis or any other disease related to alterations in an organism.
The epigenetic modifications and/or information are propagated trans-generationally to daughter cells through multiple somatic cell divisions. An organism’s genome can be modified by various chemical compounds or species in the biological system leading to changes in gene expression. These modifications are called the epigenome.
EPIGENETICS

Genetics vs. Epigenetics

Genes don't dictate our health. Genes load the gun, but environment pulls the trigger.

Much less is known about the epigenetic inheritance system than traditional genetics. Genetics is based on cell lineages and clonal inheritance. Gametogenesis produces single haploid cells that fuse to form a diploid zygote. The organism thus starts as a single cell and ends up as a clone of cells. If a mutation or chromosomal change occurs in a somatic cell, then all its descendants would be expected to have the same genotype.
In contrast, epigenetic changes often occur in groups of cells, for example, the induction of muscle tissue in mesoderm cells. This is due to a specific signal which impinges on a group of cells with the same receptor. Some epigenetic events are clonal, and X chromosome inactivation is an excellent example.
Genetic changes are stable and rarely reversed, whereas epigenetic changes are often reversed.
Environmental influences do not change the genotype (leaving aside mutagens), and there is no inheritance of acquired characteristics. Epigenetics is quite different, because normal development depends on communication between cells. Thus, a hormone, morphogen or growth factor may induce an epigenetic change that may be heritable. This means that the environment of a cell may be all important in determining its properties or its fate in the developing organism.
It is said that, “Genes don't dictate our health. Genes load the gun, but environment pulls the trigger.” Epigenetics is that environment. Changes in the internal and external environment of a biological system, such as nutritional changes, oxidative and nitrosative stress, toxic exposures, drugs, and social influences lead to epigenetic alterations.
Epigenetics are extremely important throughout one’s lifespan, but particularly during prenatal development. When a new human embryo is formed, roughly 99% of the epigenetic changes acquired during the lifetime of the parents are reprogrammed. This allows the cells of the developing embryo to accrue their own epigenome and differentiate into all the different tissues found in the fully formed organism.
Epigenetic patterns or signatures change throughout our lifetime. Our environment, nutrition, psychoemotional experiences, and toxic exposures can trigger epigenetic changes that modify and shape our phenotype. These changes may have a positive physiological or evolutionary impact, allowing us to adapt to our environment, or they may be considered harmful, leading to processes that make us more vulnerable to disease and affect our well-being.
Despite having the same DNA, different cell types have distinct gene expression (mRNA) patterns to perform different functions. One mechanism of this differential gene expression is through epigenetic changes, which some have argued may also explain some of the variation in behavioral phenotypes of humans. One key aspect of the “epigenome” is that, unlike the DNA sequence, it may be modified by environmental, dietary and even pharmaceutical interventions.
In other words, epigenetics, in a broad sense, is a bridge between genotype and phenotype - a phenomenon that changes the outcome of a locus or chromosome without changing the underlying DNA sequence. A given genotype can confer a variety of phenotypes in the presence of different environmental factors. This ability is called “phenotypic plasticity” and refers to some of the changes in an organism's behavior, morphology and physiology in response to its unique environment. Modifications in gene expression are controlled by fundamental epigenetic mechanisms including DNA methylation, histone modifications, chromatin remodeling and microRNAs that act as regulatory molecules.
Epigenetic modifications are of particular interest in the field of cancer research since their impact on the epigenome is involved in cell proliferation, differentiation and survival. Many other diseases, such as neurodegenerative disorders, are also associated with epigenetic alterations. At least half of all tumor suppressor genes are inactivated through epigenetic mechanisms in tumorigenesis.
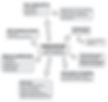
The above diagram depicts primary mechanisms involved in epigenetic changes that affect modifications in gene expression:
-
DNA methylation
-
Histone modifications
-
Non-coding RNAs (microRNAs)
These mechanisms regulate gene expression as well as various cellular and biological functions related to homeostasis, allostasis and disease.
Epigenetic mechanisms provide an interface by which the environment influences gene activity. In many diseases, including all cancers, the epigenetic control of the genome is heavily distorted. The following is a description of the three epigenetic mechanisms:
DNA methylation is a chemical process that adds a methyl group (-CH3) to DNA. It is highly specific and always happens in a region in which a cytosine-nucleotide is located next to a guanine nucleotide that is linked by a phosphate; this is called a CpG site. DNA methylation is the most widely studied form of epigenetic modification and occurs within the one-carbon metabolism pathway, which is dependent upon several enzymes in the presence of dietary micronutrients as cofactors, including the availability of folate, choline, and betaine through the diet. Methyl groups are acquired via a specific metabolic pathway that involves important vitamins like folate, B12, and riboflavin. Thus, a deficiency of these dietary factors and exposures to toxic chemicals in the environment can increase the risk of a variety of diseases.
Through an ATP-driven reaction methionine is converted into S-adenosylmethionine (SAM), the universal cellular methyl donor. DNA methyltransferases (DNMTs) covalently attach methyl groups from SAM to the carbon-5 position of cytosine bases, generating 5-methylcytosine thus methylating DNA. In the context of nutritional biochemistry, it is significant that the one-carbon metabolism pathway is cyclical and is regenerated via dietary micronutrients.
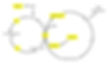
Substrates obtained via diet are highlighted in yellow. Vitamin B6 is a cofactor to serine hydroxymethyltransferase in the conversion of tetrahydrofolate (THF) to 5,10-methylene THF. Vitamin B2 is a precursor to FAD, which is a cofactor to methylenetetrahydrofolate reductase (MTHFR) in the conversion of 5,10-methylene THF to 5-methyl THF. Vitamin B12 is a precursor to methionine synthase, involved in the production of methionine from homocysteine and betaine. Acronyms: dihydrofolate (DHF), flavin adenine dinucleotide (FAD), dimethylglycine (DMG), methylenetetrahydrofolate reductase (MTHFR), S-adenosylhomocysteine (SAH), tetrahydrofolate (THF).
Methylenetetrahydrofolate reductase (MTHFR) is crucial to one-carbon metabolism, catalyzing the conversion of homocysteine to methionine and generating 5-methyltetrahydrofolate. Because of this relationship, the role of MTHFR genotype in DNA methylation has been thoroughly investigated.
Multiple processes, including gene expression, X-chromosome inactivation, imprinting, chromatin organization and other biological processes are controlled by DNA methylation. Proper methylation is vital in prenatal development after reprogramming has occurred, playing a key role in tissue differentiation. The addition of a methyl group to cytosine frequently occurs at gene promoter regions with CpG islands, which are regions of large repetitive CpG dinucleotides occupying 60% of the promoter region. Methylation of CpG dinucleotide(s) has been associated with disease states including cancer. The enzymes responsible for DNA methylation are the DNA methyltransferases, which currently are categorized into five classes based on their specific enzymatic and physiological functions.
The process of methylation occurs through the transfer of a methyl group to a histone from adenosyl methionine (AdoMet), and S-adenosylhomocysteine (AdoHcy) inhibits the action of DNMTs. AdoHcy hydrolase can hydrolyze AdoHcy into adenosine and homocysteine, and therefore, could be employed as a therapeutic agent for epigenetic diseases.

This image shows a DNA molecule that is methylated on both strands on the center cytosine.
Image by Christoph Bock of CeMM Research Center for Molecular Medicine of the Austrian Academy of Sciences, Vienna.
Histones are proteins that are the primary components of chromatin, which is the complex of DNA and proteins that makes up chromosomes. Histones act as a spool around which DNA can wind. When histones are modified after they are translated into protein (i.e., post-translation modification), they can influence how chromatin is arranged, which, in turn, can determine whether the associated chromosomal DNA will be transcribed. If chromatin is not in a compact form, it is active, and the associated DNA can be transcribed. Conversely, if chromatin is condensed (creating a complex called heterochromatin), then it is inactive, and DNA transcription does not occur.
There are two main ways histones can be modified: acetylation and methylation. These are chemical processes that add either an acetyl or methyl group, respectively, to the amino acid lysine that is located in the histone. Acetylation is usually associated with active chromatin, while deacetylation is generally associated with heterochromatin. The acetylation of histone proteins at various amino acid residues is regulated by histone acetyltransferases (HATs) and histone deacetylases (HDACs). The process of methylation occurs through the transfer of a methyl group to a histone from adenosyl methionine (AdoMet), and S-adenosylhomocysteine (AdoHcy) inhibits the action of DNMTs. AdoHcy hydrolase can hydrolyze AdoHcy into adenosine and homocysteine, and therefore, could be employed as a therapeutic agent for epigenetic diseases.
Recently, it has become evident that RNA, particularly noncoding RNAs, have a hand in controlling multiple epigenetic phenomena. Genes can also be turned off (silenced) by RNA when it is in the form of antisense transcripts, noncoding RNAs, or RNA interference. RNA might affect gene expression by causing heterochromatin to form, or by triggering histone modifications and DNA methylation. Non-coding RNAs make up the vast majority of the human genome and play a regulatory role in the expression of our genes. The most well understood of these is microRNA. These roughly 22-nucleotide-long regions are believed to help regulate more than half of our protein-coding genes by reducing the transcription of proteins, thus holding a place of great importance in normal functioning of the cell and in disease.
While epigenetic changes are required for normal development and health, they can also be responsible for some disease states. Disrupting any of the three systems that contribute to epigenetic alterations can cause abnormal activation or silencing of genes. Such disruptions have been associated with cancer, syndromes involving chromosomal instabilities, and mental retardation.
Epigenetic Therapy
The fact that many human diseases, including cancer, have an epigenetic etiology has encouraged the development of a new therapeutic option that might be termed “epigenetic therapy”. Many agents have been discovered that alter methylation patterns on DNA or the modification of histones in either a positive or negative way (see diagram below).

Dietary factors have become agents of strong interest in the field of epigenetics. Several bioactive dietary components which exhibit potential to both prevent and treat disease have been identified and researched. In fact, several naturally occurring dietary phytochemicals have been demonstrated to have anticarcinogenic properties and have been shown to play a role in regulating biological processes. Studies have shown that natural products have epigenetic targets in cancer cells and can act as cancer preventive agents. Compounds found in dietary phytochemical preparations such as teas, garlic, soy products, herbs, grapes and cruciferous vegetables are now generally accepted to defend against the development of many different types of tumors as well as to act as epigenetic modulators that impact not only the initiation, but also the progression of diseases including cancer.
Epigenetics and Dietary Factors (Nutri-epigenetics)
One of the best examples of this is polyphenols that are present in fruits and vegetables. These classes include: flavonoids, stilbenes, phenolic acids, benzoquinones, acetophenones, lignins and xanthones. The chemo-preventative potential of dietary polyphenols can be traced to their ability to inhibit DNMTs as well as their ability to act as histone modifiers. Both of these properties of dietary polyphenols can significantly change the epigenome of cancer cells and are being used clinically as anticancer agents.
Polyphenolic compounds in green tea may actively reduce the risk of diseases such as cancer. One subcategory of polyphenols, catechins, is the most abundant of the bioactive compounds in green tea. These include (−)-epicatechin (EC), (−)-epicatechin-3-gallate (ECG), (−)-epigallocatechin (EGC), and EGCG. While all of the aforementioned catechins have been found to share similar properties, the most efficient of these compounds in targeting factors like DNMTs is EGCG. EGCG accounts for more than 50% of the active compounds in green tea and has been extensively studied for its anticarcinogenic properties. An increasing number of studies have shown a positive correlation between the consumption of EGCG and the inhibition of oral, breast, prostate, gastric, ovarian, esophageal, skin, colorectal, pancreatic, and head and neck cancers.
Another dietary polyphenol is resveratrol which is naturally found in several plants including peanuts, grapes, mulberries, cranberries and blueberries, and Polygonum cuspidatum or Japanese knotweed but is most abundant in the skin of grapes. Resveratrol acts as an antioxidant and inhibits the oxidation of low density lipoproteins, the aggregation of platelets, and eicosanoid synthesis and induces platelet nitric oxide production, which may help protect against atherosclerosis and coronary heart disease. It also acts as an anti-inflammatory agent by inhibiting cyclooxygenase activity and by releasing cytokines from macrophages in chronic obstructive pulmonary disease. Antiproliferative properties of resveratrol have been reported in liver, skin, breast, prostate, lung and colon cancer cells.
One of the most exciting dietary polyphenol rich plants is Curcuma longa. Curcumin is the main component of the spice turmeric and is responsible for the yellow pigmentation of curry. This bioactive dietary component appears to have anti-inflammatory, antioxidant, antiangiogenic and anti-cancer properties and is used as a therapeutic agent in Ayurvedic Indian and traditional Chinese medicine. Investigations indicate that curcumin inhibits DNMT activity by covalently blocking the catalytic thiolate of C1226 of DNMT1. Moreover, there is evidence that curcumin may be an effective DNA hypomethylating agent that could facilitate the expression of inactive pro-metastatic and proto-oncogenes. Curcumin also has epigenomic effects in that genomic DNA from leukemia cells show global hypomethylation after curcumin treatments.
Polyphenols
Isothiocyanates

by Harika Deng for PP Design
Folate
Dietary folate is the most extensively studied micronutrient in animal and epidemiological DNA methylation research. Folate is reduced to dihydrofolate (DHF) and subsequently to tetrahydrofolate (THF), serving as a single carbon donor in the form of 5-methyl THF.
Consequently, 5-methyl THF feeds into the one-carbon metabolism cycle by donating its methyl group to homocysteine converting it to methionine. Cofactor B vitamins provide the enzymatic support necessary for these transformations, making it possible for dietary folate to feed into the one-carbon metabolism cycle to replenish cellular SAM.
For this reason, folate supplementation has generally been associated with increased DNA methylation and vice versa for folate restriction.
The water-soluble vitamins B2, B6, and B12 have an important catalytic role in folate and one-carbon metabolism. Vitamin B6 serves as a coenzyme to serine hydroxymethyltransferase, the key enzyme in the folate cycle converting THF to 5,10-methylene THF.
Riboflavin, or vitamin B2, is a precursor for flavin adenine dinucleotides (FAD), which is a cofactor to MTHFR, the enzyme responsible in the reduction of 5,10-methylene THF to 5-methyl THF.
Vitamin B12 is the coenzyme of methionine synthase, which catalyzes the reaction of homocysteine, the by-product of SAM, to methionine. Thus, dietary consumption of these water-soluble B vitamins has the potential to affect the efficiency of the one-carbon metabolism pathway.
Vitamins B2, B6, and B12
Choline is an indirect methyl group donor for one-carbon metabolism. Within this pathway, dietary choline is oxidized to betaine. Betaine then contributes to methionine homeostasis through the donation of a methyl group to homocysteine, resulting in homocysteine’s conversion to methionine. Thus, several animal in vivo (and, to a lesser extent, human studies) have investigated the role of dietary choline and/or betaine and their impact on gene DNA methylation.
Numerous other dietary nutrients and compounds have also been found to have positive epigenetic targets. As the field of nutri-epigenetics continues to emerge, it will enable clinical and public health practices to apply epigenetically-driven therapeutic and preventative strategies when evaluating a population or individuals in a certain disease state. Nutritional recommendations based upon individual epigenetic profiles are being clinically used to prevent and treat illness.
Choline and Betaine
Early life experiences exert a profound and long-lasting influence on physical and mental health throughout life. The efforts to identify the primary causes of this have significantly benefited from studies of the epigenome - a dynamic layer of information associated with DNA that differs between individuals and can be altered through various experiences and environments. Understanding the mechanisms involved in the initiation, maintenance, and heritability of epigenetic states is thus an important aspect of research in current biology, particularly in the study of learning and memory, emotion, and social behavior in humans.
Moreover, epigenetics in psychology provides a framework for understanding how the expression of genes is influenced by experiences and the environment to produce individual differences in behavior, cognition, personality, and mental health.
Research now shows how diverse social and environmental factors (the social determinants of health) such as maternal health and education, nutrition, environmental toxins, social conditions such as housing and poverty, and child rearing practices affect how our genetic building blocks (DNA) are expressed.
The differences in gene expression contribute to individual differences in health, development and behavior. Social epigenetics is the process by which early life experiences influence chemical reactions that in turn alter the ways our genes function or are expressed. And these differences in expression influence lifelong health and wellbeing.
The term "social determinant of health" often refers to any nonmedical factor directly influencing health, including: values, attitudes, knowledge, and behaviors. However, it can also refer to more external sources of influence such as family, neighborhood and social network context. A large and convincing body of literature over the last several decades shows that health across one's lifespan is strongly linked to social disadvantage.
For example, neighborhoods can influence health through their physical and geographic characteristics, such as air and water quality, lead paint exposure, proximity to both health promoting and suppressing features (i.e. hospitals and nutritious food stores vs. toxic factories and fast food), access to green space, and so on. Additionally, more social aspects of neighborhoods such as strong social cohesion show far better health and safety.
Substantial evidence indicates that pathways initiated by childhood social adversity can be interrupted. Studies show that high-quality early development interventions - including center-based programs to nurture and stimulate children and to support and educate parents - greatly ameliorate the effects of social disadvantage on children’s cognitive, emotional/behavioral, and physical development; the first five years of life appear to be most crucial, although opportunities for intervention continue throughout childhood and adolescence.
In short, social environment, particularly social deprivation is associated with a wide range of epigenetic change in children and young adults. But more research exploring the overlap between social and natural environmental links to epigenetics and health is desperately needed.
Social Epigenetics
Personalized medicine is the application of an individual’s personal genetic profile to predict disease, prevent disease through medical interventions, and make decisions about lifestyle and disease management based on the needs of each individual patient. Moreover, genetic screening is important for the personalization of treatment for a patient.
In conventional western medicine, patients are treated by a “one-size-fits-all” approach within a standard pharmaceutical protocol. Treatment decisions are mainly based on the clinical stage of the disease and the patient’s medical and family history - ignoring the individual’s underlying biology.
While this approach has achieved some therapeutic efficacy in managing certain diseases and cancers (e.g., testicular cancers, and pediatric leukemias), the overall survival rate of cancer patients has improved little in the last decades, arguing for the need of novel ideas and strategies in disease management.
The new epigenetic treatment approach should integrate comprehensive disease biology and individualized multi-therapeutics into other personal attributes (age, sex, nutrition, psychological conditions and gut microbiome).
The epigenetics arena has become a rich and exciting global research environment, inspiring collaborations between commercial and academic partners that have identified many significant epigenetic biomarkers for a wide range of serious and debilitating diseases.
Precise mapping and measurement of epigenetic biomarkers will continue to improve clinical practice by enabling diagnosis during early stages of disease development, even before genetic changes have taken place. Epigenetic-based diagnostics that can detect early disease signals will provide opportunities for clinical intervention before symptom progression has impacted on quality of life - when patients are still relatively healthy, and conditions favor treatment success.
These highly-specific biomarkers can help to personalize disease management that allows treatment response to be monitored more precisely. Nutritional and lifestyle interventions may then be adjusted accordingly to optimize treatment outcomes.
Unfortunately, a significant drawback to the prevailing approach to personalized medicine is its primary or myopic focus on pharmaceuticals and technology-driven diagnostics rather than on basic preventive public health measures. Abundant evidence exists that healthy nutritional choices, reducing personal or collective toxic exposures, exercise, and stress reduction, increase general health much more efficiently than pharmaceutical treatment of disease.
Epigenetics offers great opportunities to improve both healthcare and, more proactively, health in general. However, the full potential of personalized medicine will remain untapped if current research stays focused in pharmaceutical intervention rather than prevention with nutrition, lifestyle and social modifications.
Most importantly, embracing the impact of the environment on health will require a new framework to guide both research and its application, and to steer public investment and research efforts toward more truly cost-efficient approaches to personalized health, healthcare and quality of life.
Personalized Medicine and Epigenetic Biomarkers
References
• Adler NE, Ostrove JM. Socioeconomic status and health: what we know and what we don’t. Annals of the New York Academy of Sciences. 1999;896(1):3–15.
• Anderson, Olivia S., Karilyn E. Sant, and Dana C. Dolinoy. Nutrition and epigenetics: an interplay of dietary methyl donors, one-carbon metabolism and DNA methylation. The Journal of nutritional biochemistry 23, no. 8 (2012): 853-859.
• Bock C. et al. Quantitative comparison of DNA methylation assays for biomarker development and clinical applications. Nature Biotechnology, June 2016 DOI: 10.1038/nbt.3605
• Braveman P, Egerter S, Williams DR. The social determinants of health: coming of age. Annual Review of Public Health. 2011;32:381–398.
• Campbell F, Conti G, Heckman JJ, et al. Early childhood investments substantially boost adult health. Science (New York, N.Y.) 2014 Mar 28;343(6178):1478–1485. [PMC free article]
• Chen P-N, Chu S-C, Kuo W-H, Chou M-Y, Lin J-K, Hsieh Y-S. Epigallocatechin-3 gallate inhibits invasion, epithelial-mesenchymal transition, and tumor growth in oral cancer cells. J Agric Food Chem. 2011;59(8):3836–3844.
• Chowdhury S, Erickson SW, Macleod SL, et al. Maternal genome-wide DNA methylation patterns and congenital heart defects. PLoS ONE. 2011;6(1):e16506. [PMC free article]
• De Kok T, Van Breda S, Manson M. Mechanisms of combined action of different chemopreventive dietary compounds. Eur J Nutr. 2008;47:51–59.
• Doi A, Park I-H, Wen B, et al. Differential methylation of tissue- and cancer-specific CpG island shores distinguishes human induced pluripotent stem cells, embryonic stem cells and fibroblasts. Nat Genet. 2009;41(12):1350–1353. [PMC free article]
• Egger, Gerda, Gangning Liang, Ana Aparicio, and Peter A. Jones. Epigenetics in human disease and prospects for epigenetic therapy. Nature 429, no. 6990 (2004): 457.
• Feinberg AP, Ohlsson R, Henikoff S. The epigenetic progenitor origin of human cancer. Nat Rev Genet. 2006;7(1):21–33.
• Fowke JH. Head and neck cancer. A case for inhibition by isothiocyanates and indoles from cruciferous vegetables. Eur J Cancer Prev. 2007;16(4):348–356.
• Goel A, Aggarwal BB. Curcumin, the golden spice from Indian saffron, is a chemosensitizer and radiosensitizer for tumors and chemoprotector and radioprotector for normal organs. Nutr Cancer. 2010;62(7):919–930.
• Goldberg, Aaron D., C. David Allis, and Emily Bernstein. Epigenetics: a landscape takes shape. Cell 128, no. 4 (2007): 635-638.
• Grafodatskaya D, Chung B, Szatmari P, Weksberg R. Autism spectrum disorders and epigenetics. J Am Acad Child Adolesc Psychiatry. 2010;49(8):794–809.
• Graham HN. Green tea composition, consumption, and polyphenol chemistry. Prev Med. 1992;21(3):334–350.
• Hassan YI, Zempleni J. Epigenetic regulation of chromatin structure and gene function by biotin. J Nutr. 2006;136(7):1763–1765. [PMC free article]
• Heerboth et al. Use of Epigenetic Drugs in Disease: An Overview. Genetics & Epigenetics 2014:6 9–19.
• Howells LM, Moiseeva EP, Neal CP, et al. Predicting the physiological relevance of in vitro cancer preventive activities of phytochemicals. Acta Pharmacol Sin. 2007;28(9):1274–1304.
• Kim JW, Amin AR, Shin DM. Chemoprevention of head and neck cancer with green tea polyphenols. Cancer Prev Res. 2010;3(8):900–909. [PMC free article]
• Knekt P, Järvinen R, Seppänen R, et al. Dietary flavonoids and the risk of lung cancer and other malignant neoplasms. Am J Epidemiol. 1997;146(3):223–230.
• Kürbitz C, Heise D, Redmer T, et al. Epicatechin gallate and catechin gallate are superior to epigallocatechin gallate in growth suppression and anti-inflammatory activities in pancreatic tumor cells. Cancer Sci. 2011;102(4):728–734.
• Link A, Balaguer F, Goel A. Cancer chemoprevention by dietary polyphenols: promising role for epigenetics. Biochem Pharmacol. 2010;80(12):1771–1792. [PMC free article]
• Loi, Michele, Lorenzo Del Savio, and Elia Stupka. Social epigenetics and equality of opportunity. Public health ethics 6, no. 2 (2013): 142-153.
• Maheshwari RK, Singh AK, Gaddipati J, Srimal RC. Multiple biological activities of curcumin: a short review. Life Sci. 2006;78(18):2081–2087.
• Maier S, Olek A. Diabetes: a candidate disease for efficient DNA methylation profiling. J Nutr. 2002;132(8):2440S–2443S.
• McGowan, Patrick O., and Moshe Szyf. The epigenetics of social adversity in early life: implications for mental health outcomes. Neurobiology of disease 39, no. 1 (2010): 66-72.
• Milagro FI, Campión J, Cordero P, et al. A dual epigenomic approach for the search of obesity biomarkers: DNA methylation in relation to diet-induced weight loss. FASEB J. 2011;25(4):1378–1389.
• Nephew KP, Huang TH-M. Epigenetic gene silencing in cancer initiation and progression. Cancer Lett. 2003;190(2):125–133.
• Sallis JF, Glanz K. The role of built environments in physical activity, eating, and obesity in childhood. The Future of Children. 2006;16(1):89–108.
• Szyf M. Early life, the epigenome and human health. Acta Paediatr. 2009 Jul;98(7):1082–1084.
• Tollefsbol, Trygve O. Dietary epigenetics in cancer and aging. In Advances in nutrition and cancer, pp. 257-267. Springer, Berlin, Heidelberg, 2014.
• Toraño et al. The Impact of External Factors on the Epigenome: In Utero and over Lifetime Biomed Res Int. 2016; 2016: 2568635.
• Tu S-H, Ku C-Y, Ho C-T, et al. Tea polyphenol (−)-epigallocatechin-3-gallate inhibits nicotine- and estrogen-induced α9-nicotinic acetylcholine receptor upregulation in human breast cancer cells. Mol Nutr Food Res. 2011;55(3):455–466.
• vel Szic, Katarzyna Szarc, Ken Declerck, Melita Vidaković, and Wim Vanden Berghe. From inflammaging to healthy aging by dietary lifestyle choices: is epigenetics the key to personalized nutrition? Clinical epigenetics 7, no. 1 (2015): 33.
• Waddington CH. The epigenotype. Endeavour. 1942;1:18–20.
Epigenetics
Epigenetics
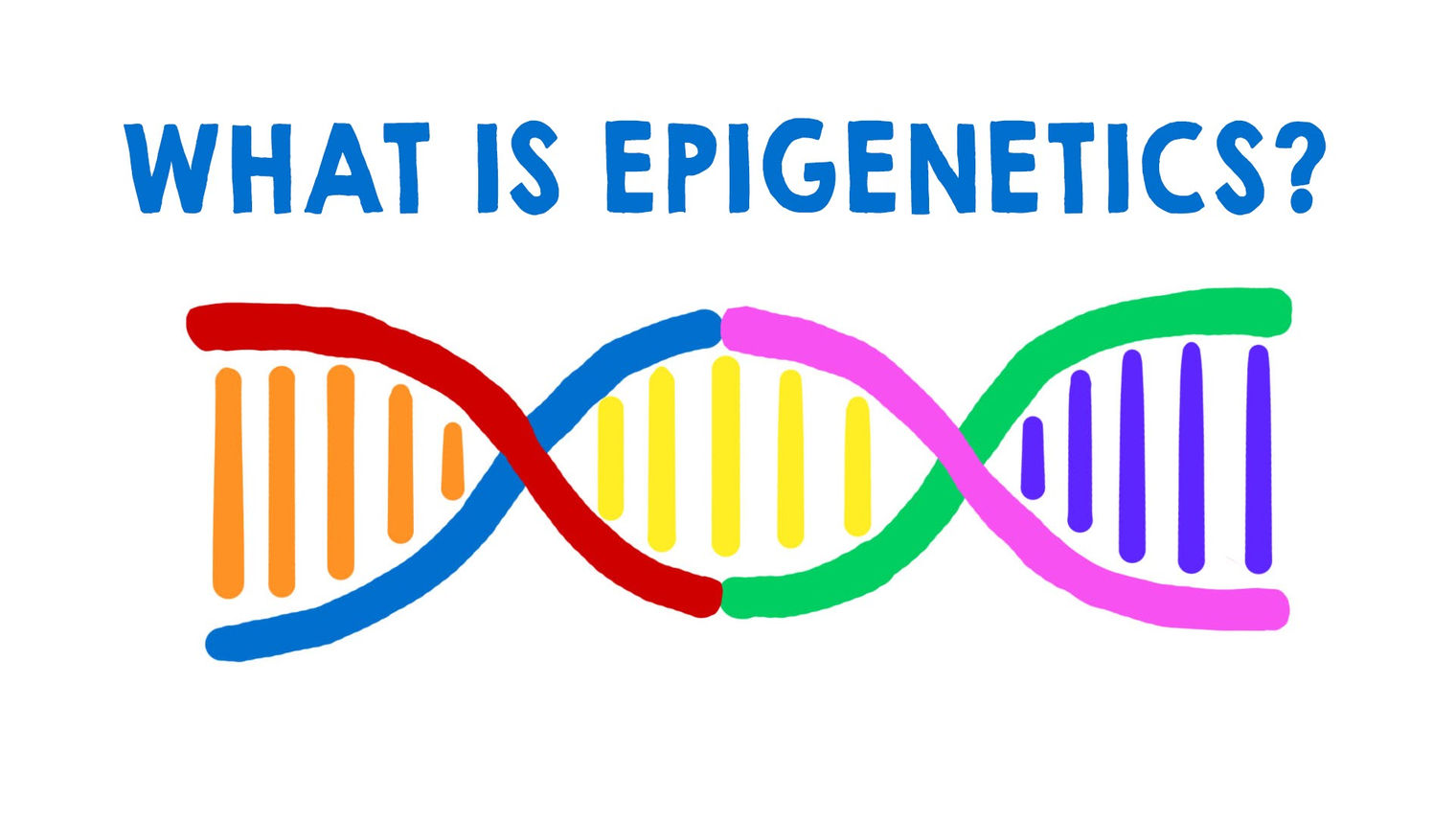

Bruce Lipton, Ph.D. Epigenetics: The science of Human Empowerment

Epigenetics - An Introduction
